
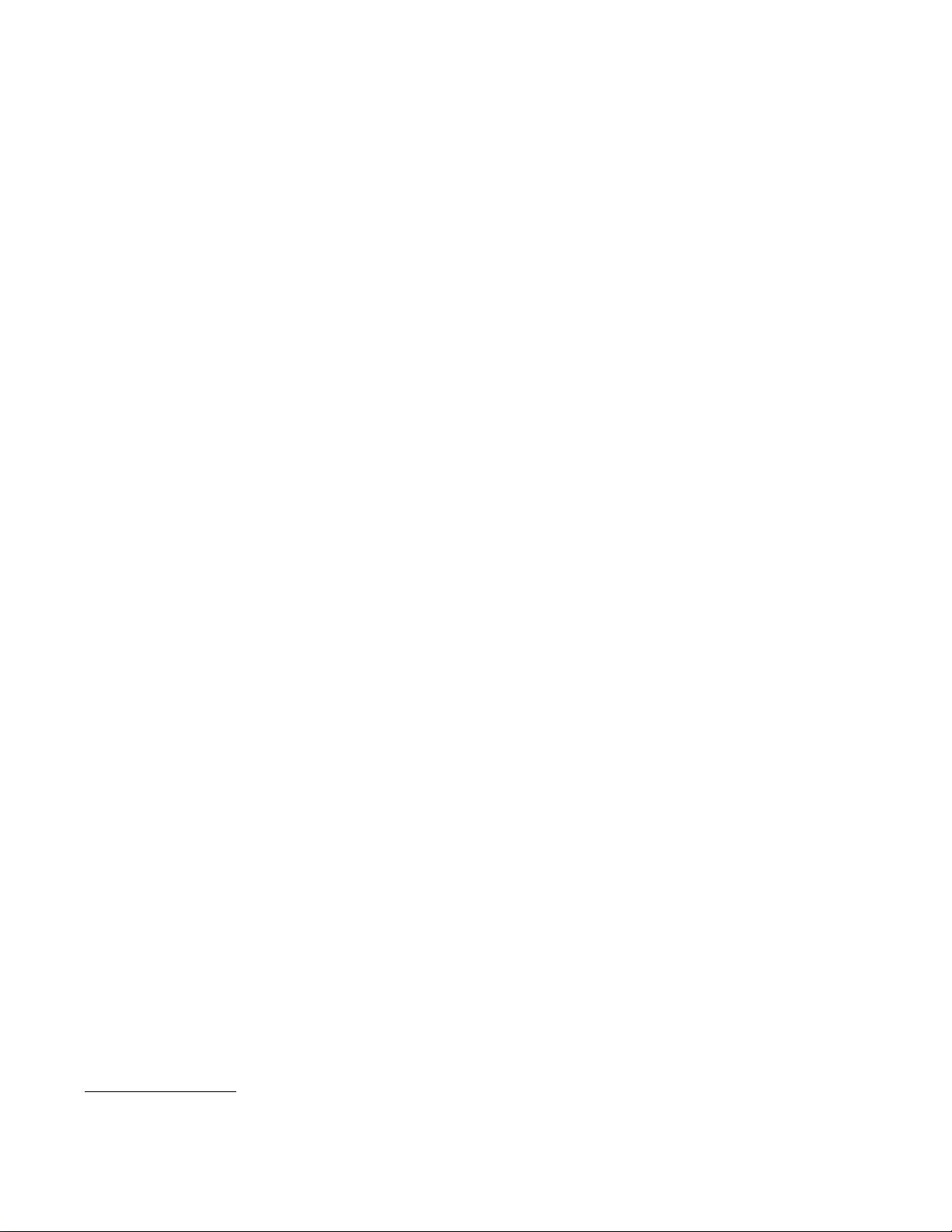



read more
Given Γ and p ∈ N∗+, let φ be a function that randomly partitions Γ into ηp = η / p mini-batches { X(1), ...,X(ηp) } , where, for i ∈ J1, ηpK, X(i) ∈ Rm×p.
The position along z is coded with a fixed-length code and, for each pair (x, y), the number of non-zero coefficients along z is coded with a Huffman code.
The training objective is to minimize the mean squared error between these cropped images and their reconstruction plus l2-norm weights decay.
Max-pooling is a core component of neural networks [11] that downsamples its input representation by appling a maxfilter to non-overlapping sub-regions.
Each layer i ∈ J1, 4K consists in convolving the layer input with the bank of filters W(i), adding the biases b(i) and applying a mapping g(i), producing the layer output.
η∑ i=j ‖Zj‖0 ≤ γ × n× η(4)(4) is solved by Algorithm 2 which alternates between sparse coding steps that involve WTA OMP and dictionary updates that use stochastic gradient descent.
The non-zero coefficients are uniformly quantized over 8-bits and coded with a Huffman code while their position is coded with a fixed-length code.
for WTA OMP only, the number of non-zero coefficients of the sparse decomposition of each patch over D is coded with a Huffman code.
For each j ∈ J1, pK,Yj = OMP (Xj ,D, k) (1) The author= fγ (Y) (2)For each j ∈ J1, pK,Zj = min z∈Rn ‖Xj −Dz‖22 st.supp (z) = supp (Ij) (3)Output: Z ∈ Rn×p.4.
For instance, [20] proves that removing a maxpooling layer and increasing the stride of the previous convolution, as the authors do, harms neural networks.
CONCLUSIONS AND FUTURE WORKThe authors have shown that, SWTA AE is more adaptated to image compression than auto-encoders as it performs variable rate image compression for any size of image after a single training and provides better rate-distortion trade-offs.
Gary J. Sullivan, Jim M. Boyce, Ying Chen, Jens-Rainer Ohm, C. Andrew Segal, and Anthony Vetro, “Standardized extensions of high efficiency video coding (HEVC),” IEEE Journal of Selected Topics in Signal Processing, vol. 7 (6), pp. 1001–1016, December 2013.[8]
it keeps the γ × n × p coefficients with largest absolute value for the nlength sparse representation of the p patches and sets the rest to 0, see (2).
Given Γ, k < m and γ ∈ ]0, 1[, the dictionary learning problem is formulated as (4).min D,Z1,...,Zη1η η∑ j=1 ‖Γj −DZj‖22st. ∀j ∈ J1, ηK, ‖Zj‖0 ≤ kst.