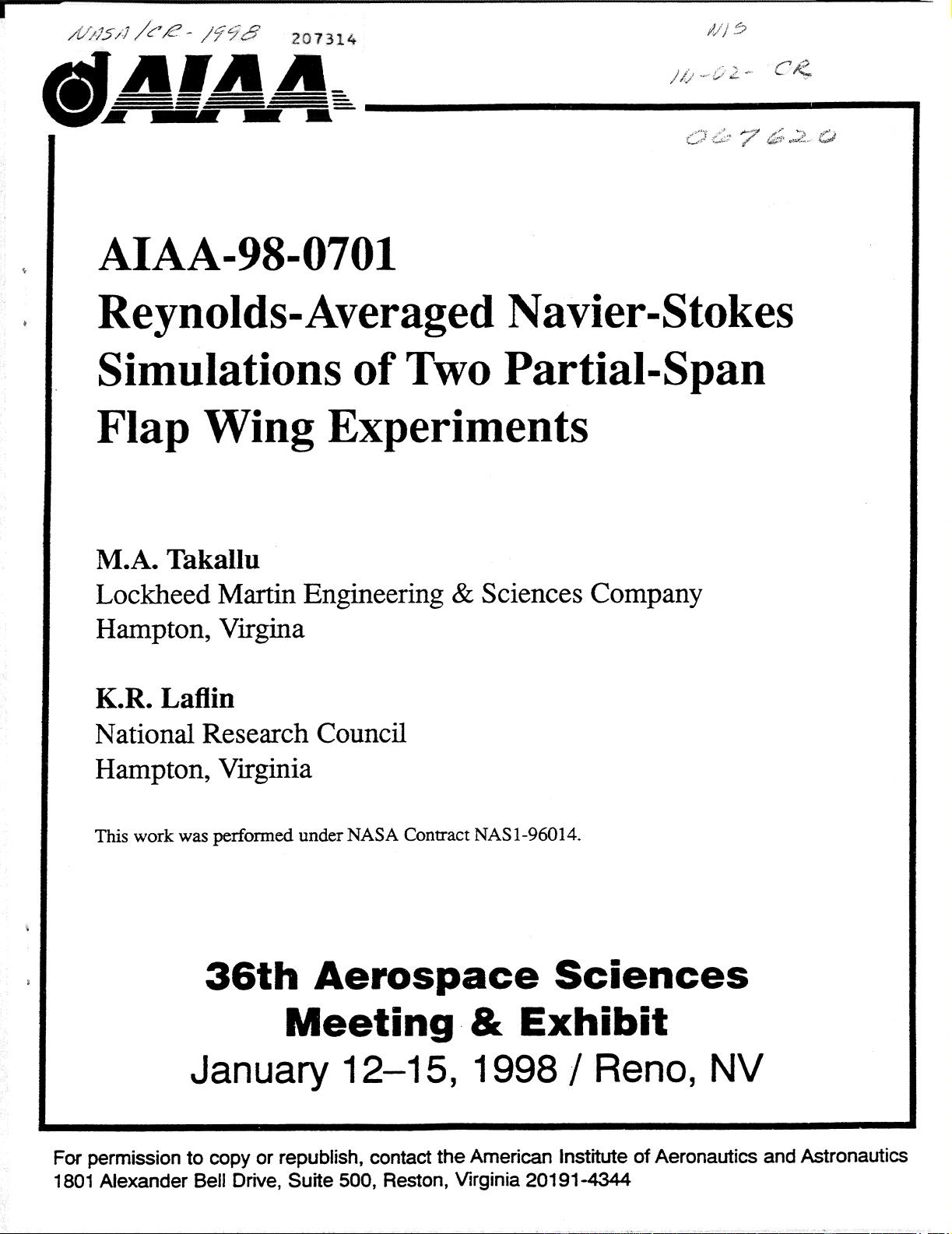
/Z> ......._,-2.- c.........._
AIAA-98-0'701
Reynolds-Averaged Navier-Stokes
Simulations of Two Partial-Span
Flap Wing Experiments
M.A. Takallu
Lockheed Martin Engineering & Sciences Company
Hampton, Virgina
K.R. Laflin
National Research Council
Hampton, Virginia
This work was performed under NASA Contract NAS 1-96014.
36th Aerospace Sciences
Meeting & Exhibit
January 12-15, 1998 / Reno, NV
For permission to copy or republish, contact the American Institute of Aeronautics and Astronautics
1801 Alexander Bell Drive, Suite 500, Reston, Virginia 20191-4344
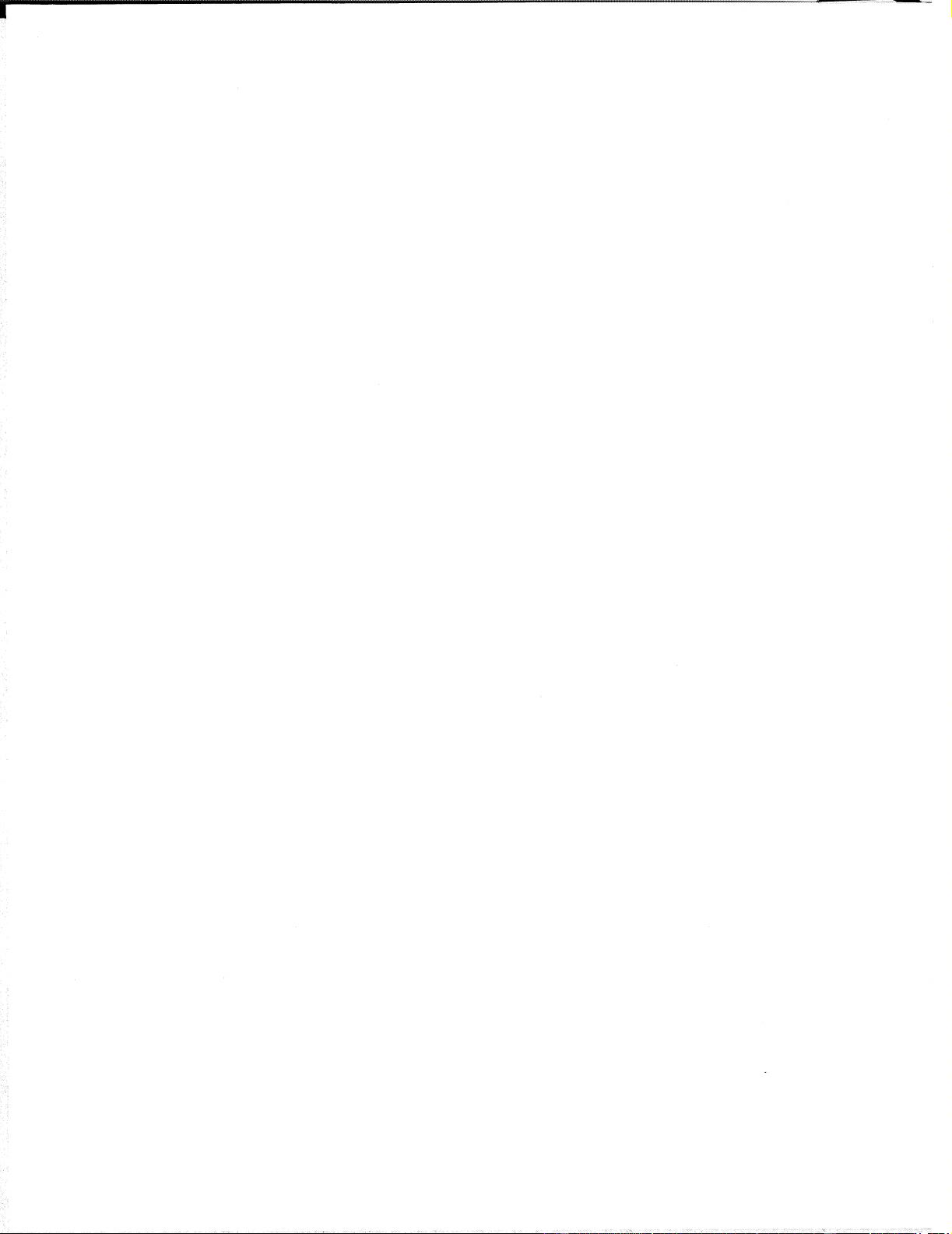

AIAA-98-0701
REYNOLDS-AVERAGED NAVIER-STOKES SIMULATIONS OF
TWO PARTIAL-SPAN FLAP WING EXPERIMENTS
M.A. Takallu*
Lockheed Martin Engineering & Sciences Company
Hampton, VA
Kelly R. Latin t
National Research Council
Hampton, VA
Abstract Introduction
Structured Reynolds Averaged Navier-Stokes
simulations of two partial, span flap wing experiments
were performed. The high-lift aerodynamic and
aeroacoustic wind-tunnel experiments were conducted
at both the NASA Ames 7- by 10-Foot Wind Tunnel and
at the NASA Langley Quiet Flow Facility. The purpose
of these tests was to accurately document the acoustic
and aerodynamic characteristics associated with the
principle airframe noise sources, including flap side-
edge noise. Specific measurements were taken that can
be used to validate analytic and computational models
of the noise sources and associated aerodynamics for
configurations and conditions approximating fight for
transport aircraft. The numerical results are used to both
calibrate a widely used CFD code, CFL3D, and to
obtain details of flap side-edge flow features not
discernible from experimental observations. Both
experimental set-ups were numerically modeled by
using multiple block structured grids. Various
turbulence models, grid block-interface interaction
methods and grid topologies were implemented.
Numerical results of both simulations are in excellent
agreement with experimental measurements and flow-
visualization observations. The flow field in the flap-
edge region was adequately resolved to discern some
crucial information about the flow physics and to
substantiate the merger of the two vortical structures, As
a result of these investigations, airframe noise modelers
have proposed various simplified models which use the
results obtained from the steady-state computations as
input.
An aerodynamic challenge related to the design of
subsonic transport aircraft is the determination and
reduction of radiated airframe noise. Research1
indicates that, as aircraft engines become quieter in the
near future, a significant contributor to airframe noise of
an aircraft on approach will be the high-lift system.
Within the complex three-dimensional flow field of a
high-lift system, the side-edge region of a partial-span
flap is suspected to produce the dominant noise source. 2
The noise associated with this region is believed to be
due to the formation and shedding of a flap side-edge
vortex and its interaction with the free shear layer.
Although the general mechanisms of flap side-edge
vortex formation are well known, details of the flow
field physics and associated unsteadiness required for
noise source generation in the flap side-edge region are
not completely understood. Knowledge of these details,
as well as other details of high-lift flow fields, are
crucial to the design of improved high-lift systems with
reduced airframe noise levels. Required flow field
details, that are not discernible from experimental
measurements or observations, will likely be determined
through the use of computational fluid dynamics (CFD).
Noise generation is an unsteady phenomenon, but
unsteady viscous three-dimensional flow field
computations are both time consuming and expensive.
For this reason, airframe noise modelers have proposed
various simplified models which use the results obtained
from steady-state Reynolds Averaged Navier-Stokes
(RANS) computations as input for noise source
models. 3-4 However, before the RANS results can be
confidently used in this capacity, they must be calibrated
against reliable test data.
*Supervisor, Aerodynamics Section
NASA Langley Program Office. Associate Fellow AIAA.
m.a.takallu @larc.nasa.gov
tResident Research Associate, Aero and Gas Dynamics
Division, NASA Langley Research Center. Non-Member.
k.r.laflin @larc.nasa.gov
In this paper, experimental results of two partial-
span flap wing experiments are used to calibrate the use
of a RANS flow solver for predicting high-lift flow
fields and providing the details required by airframe
noise models. Although, Jones et al 5 have demonstrated
American Institute of Aeronautics and Astronautics

theabilityof such a flow solver to simulate flow fields
about two-dimensional and three-dimensional muki-
element high-lift configurations, it is not known if
RANS flow solvers are capable of providing the small-
scale aerodynamic details associated with airframe
noise sources. 6 In the present work, structured RANS
computations were used as a modem analysis tool to
study two high-lift aerodynamic and aeroacoustic wind-
tunnel experiments in order to both calibrate a widely
used CFD code and to simulate some of the flow
features not captured by the experiments. Therefore, a
brief discussion of the two NASA experiments is
followed by a description of the numerical strategy.
Computed results, that concentrate on the flap side-edge
region, will be presented in detail and compared with
experimental data.
Experimental Investigations
A series of partial-span flap wing wind-tunnel
experiments were conducted at both the NASA Ames
Research Center 7- by 10-Foot Wind Tunnel 7 (7x10)
and at the NASA Langley Quiet Flow Facility 8 (QFF).
The purpose of these tests was to accurately document
the acoustic and aerodynamic characteristics associated
with the principle airframe noise sources, including flap
side-edge noise. Specific measurements were taken that
can be used to validate analytic and computational
models of the noise sources and associated
aerodynamics for configurations and conditions
approximating flight for transport aircraft.
7xl 0 Experiment
QFF Experiment
In an effort complementary to the 7x10 test,
aerodynamic and noise issues were investigated at the
QFF using a 16 inch chord model of similar geometry to
that of the 7x10 test model but with an aspect ratio of
2.25. In the QFF (Figure 2), jet flow enters vertically
through the floor of an anechoic chamber via a
converging inlet nozzle which accelerates the jet flow to
the desired entrance conditions. The partial-span flap
model (Figure 3) is mounted between two rectangular-
shaped end plates and positioned in the vertical jet
(Figure 4). The jet is constrained in the spanwise
direction of the wing by the two end plates but is
otherwise allowed to expand. Flow exits through a
converging exhaust nozzle positioned at the ceiling of
the large anechoic chamber. Because of the expansion of
the jet flow between the two end plates, wing loading
measurements were lower than those of the 7xl 0 test for
similar angles of attack and flap-deflection angles.
Therefore, the angles of attack of the QFF experiment
were adjusted so that the wing loadings more closely
matched those obtained in the Ames test. Microphone
arrays, directional microphones, 5-hole probe wake
surveys, pressure sensitive paint (PSP) applications, and
laser light sheet flow visualization were employed as
part of the QFF test.
Numerical Approach
All computations were performed on the Cray C-90
and Cray Y-MP super-computers of the National
Aerodynamic Simulation (NAS) facility located at the
NASA Ames Research Center.
The NASA Ames 7x10 test provided a data base of
aerodynamic forces and moments with accompanying
wing pressure distributions (750 pressure taps) for a
partial-span flap wing model 7. A sketch of the model
set-up is shown in Figure 1. The model was designed so
that a constant-chord rectangular wing with a NACA
632-215 Mod B airfoil cross-section resulted when the
flap was retracted; thereby providing a reference "two-
dimensional" flow to which the three-dimensional flows
of deployed-flap configurations could be compared. The
wing chord and span are Cw=30 inches and 2.5 c w,
respectively. The half-span flap has a chord of 0.3c w.
Measurements were taken at angles of attack ranging
from 0 to 15 degrees with flap deflections of 29 and 39
degrees. Microphone array and 7-hole probe near wake
surveys where also conducted as part of the 7xl 0 test.
Flow Solver
The RANS flow solver CFL3D 9 was used to
simulate both the 7x10 and the QFF experiments. This
code uses the unsteady, three'dimensional,
compressible, thin-layer Navier-Stokes equations to
model the flow on body-fitted structured grids with
multiple-block topologies. It is a semi-discrete cell-
centered finite-volume scheme, that can make use of
grid sequencing, local time-stepping, and multigridding
to accelerate convergence to a steady state; all three
techniques were utilized for all computations presented
herein. CFL3D allows both C0-continuous (one-to-one
matching at block interfaces) and patched block
interfaces; both of these were used in this study.
CFL3D advances the solution using an implicit
approximate factorization (AF) scheme. A block
tridiagonal inversion results for each AF sweep by using
2
American Institute of Aeronautics and Astronautics

first-orderaccurateupwind-biaseddifferencingto
approximatetheimplicitspatialderivatives.However,
becausesteadystatesolutionsareconsidered,flux-
differencesplitting(FDS)canbeusedin conjunction
with a diagonalschemeto requireonly a scalar
tridiagonalinversionfor eachAF sweep.This
simplificationreducesboththerequiredmemoryand
CPUtimewithoutdecreasingthe accuracyof the
computations.CFL3Dcanalso employflux-vector
splitting,butthisoptionwasnotusedtoperformthe
computationspresentedhere.Explicitspatialderivatives
areapproximatedusingthird-orderaccurateupwind-
biaseddifferencingfortheinviscidtermsandsecond-
orderaccuratecentereddifferencingfor theviscous
terms.Fullyturbulentsolutionswereobtainedusingthe
Spalart-Allmaras1° one-equationturbulencemodels.
The Spalart-Allmarasturbulencemodelis often
employedwhenhigh-liftconfigurationsareconsidered
becauseit hasbeenperformingadequatelyfor multiple
solidsurfacesandwake/boundarylayerinteractions.In
addition,itseemstoproducemoreaccuratesolutionsin
reverseflowregions,ascomparedwithotherturbulence
models.
Forpatchedzones,datatransferbetweenzonesis
accomplishedby linear interpolationin the
computationalcoordinatesystem.The interpolation
coefficientsrequiredforCFL3Dareobtainedasapre-
processingstepusingtheRONNIE9preprocessor.
Gridding Strategy
Since these computed high-lift flow fields are to be
directly compared with experimental measurements and
observations, it is logical, as demonstrated by Cao et
a111 that the wind tunnel walls need to be modeled as
part of the numerical simulation. The multiple
components of a high-lift system require multiple grid
blocks. As it was mentioned earlier, CFL3D allows both
C0-continuous (one-to-one matching at block
interfaces) and patched block interfaces; both of these
were used in this study. The addition of the rectangular
walls adds some difficulty to a C0-continuous grid
construction, especially when the test facility geometry
is complex, such as the geometry of the QFF. An
alternative to C0-continuous grid construction is to use
patched block interfaces, By using patched grids, the
grid construction procedure is simplified and the
required number of grid points can generally be
reduced. In order to substantiate the use of patched grid
interfaces in future studies, computations where
performed on both C0-continuous and patched grids and
the resulting solutions where compared to the
experimental data.
Numerical Simulation of 7xl O Experiment
The partial-span flap wing tested in the NASA
Ames 7x10 was modeled using both a 16-block grid
with C0-continuous interfaces (Figure 5) and a 19-block
grid with mixed C0-continuous and patched interfaces
(Figure 6). The 16-block and 19-block grids have a total
of approximately 2.3 and 2.6 million points,
respectively. In each grid, the constant-area rectangular
test section was extended 15 chord lengths upstream and
downstream from the wing. Wing angles of attack of 4,
8, and 10 degrees with a flap deflection of 29 degrees
were considered for the numerical simulations. The
Reynolds number and Mach number used for the
simulations were 3.6x10 .6 and 0.2, respectively;
matching the 7x10 test conditions. The computational
grids used viscous grid spacing suitable for turbulent
boundary-layer computations at all wing surfaces. In
addition, a high concentration of grid-points was used to
resolve the flow field about the flap side-edge. Inviscid
grid-points spacing was used at all solid surfaces, apart
from those of the wing components, to limit the total
number of grid points and, thus, the required memory
and CPU time of the simulations. This methodology was
successfully used by other researchers to compute the
above set-up both with structured 6 and unstructured
grids. 12
Numerical Simulation of QFF Experiment
When a constant area rectangular-shaped test
section is considered, the grid generation, boundary-
condition specification and computations for a
numerical simulation are relatively simple; this is the
case for the numerical simulation of the 7×10
experiment. However, numerical simulations of the QFF
experiment present several challenges that have not been
previously addressed for the calculation of three-
dimensional, viscous, high-lift flow simulations. These
challenges include the numerical simulation of a high-
lift device in a semi-constrained jet flow, the presence of
large-scale circulatory flow throughout a large test
chamber, and the existence of large regions of near-
stagnant flow which is known to cause convergence and
accuracy difficulties for compressible codes.13A series
of grid alterations, such as grid refinements around the
end-plates and inside the area representing the anechoic
room, were performed to meet these computational
challenges.
The QFF experimental set-up was modeled using a
21-blocked grid with C0-continuous interfaces having
approximately 3.2 million grid points (Figure 7). As
illustrated in Figure 7a and 7b, the first 12 grid blocks
3
American Institute of Aeronautics and Astronautics