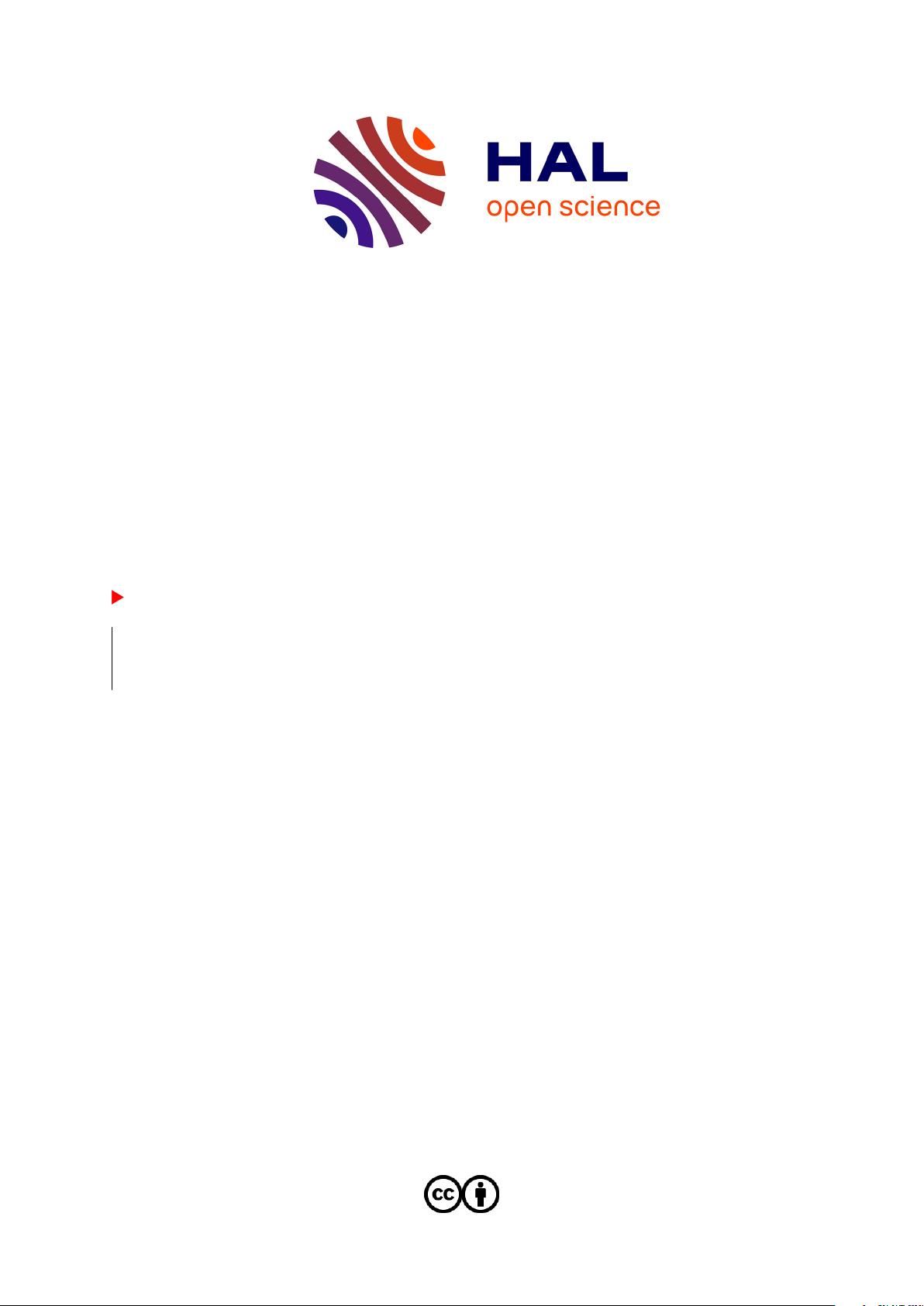



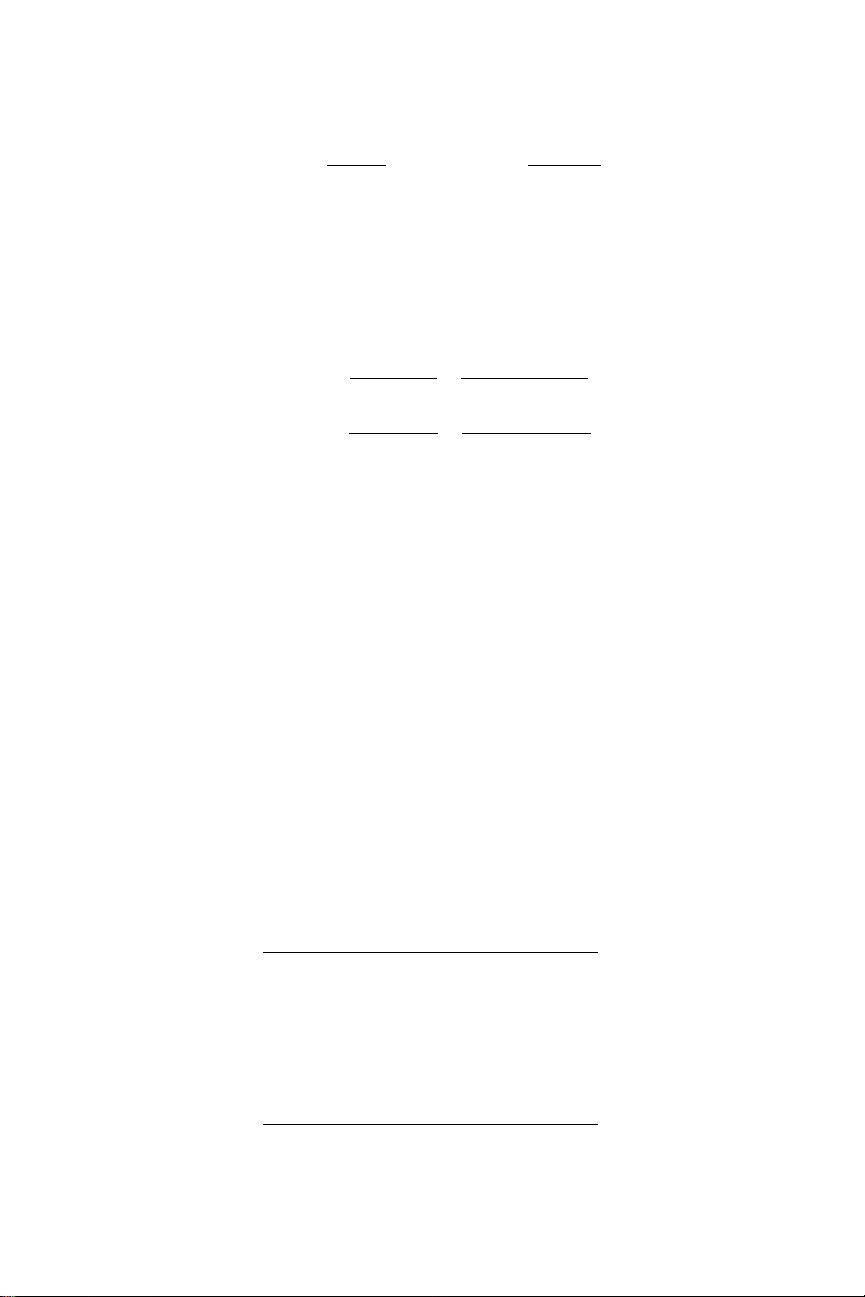
read more
Attention should also be paid to axes of symmetry: as opposed to structural boundaries where the averaging region lying outside the structure is chopped, a special averaging procedure is needed to account for material points that are not represented in the finite element model.
The most robust way of calibrating the internal length is by a semi-inverse technique which is based on computations of size effect tests.
The influence of microcracking due to external loads is introduced via a single scalar damage variable d ranging from 0 for the undamaged material to 1 for completely damaged material.
During load cycles, microcracks close progressively and the tangent stiffness of the material should increase while damage is kept constant.
In tension, microcracks are perpendicular to the tensile stress direction; in compression microcracks open parallel to the compressive stress direction.
After anincremental growth of damage, the new damage surface is the sum of two ellipsoidal surfaces: the one corresponding to the initial damage surface, and the ellipsoid corresponding to the incremental growth of damage.
This modification of the model is necessary in order to achieve consistent computations in the presence of strain localization due to the softening response of the material [8].
This model, however, enables a proper description of failure that includes damage initiation, damage growth, and its concentration into a completely damaged zone, which is equivalent to a macrocrack.
The damage energy release rate isY ¼ ÿr @c @d ¼ 1 2 eijC0ijkleklwith the rate of dissipated energy:’f ¼ ÿ @rc @d ’dSince the dissipation of energy ought to be positive or zero, the damage rate is constrained to the same inequality because the damage energy release rate is always positive.
To speed the computation, a table in which, for each gauss point, its neighbors and their weight are stored can be constructed at the time of mesh generation.
For a linear displacement interpolation, a is the solution of the following equality where the states of strain and stresses correspond to uniaxial tension:hf ¼ Gf ; with f ¼ Z 10 Z O ½ ’dð~nÞnkstklnlni njdOdeij ð24Þwhere f is the energy dissipation per unit volume, Gf is the fracture energy, and h is related to the element size (square root of the element surface in a two-dimensional analysis with a linear interpolation of the displacements).