
1
Addressing Limitations in Existing ‘Simplified’ Liquefaction Triggering
1
Evaluation Procedures: Application to Induced Seismicity in the Groningen
2
Gas Field
3
4
R.A. Green
1
, J.J. Bommer
2
, A. Rodriguez-Marek
3
, B.W. Maurer
4
,
5
P.J. Stafford
5
, B. Edwards
6
, P.P. Kruiver
7
, G. de Lange
8
, and J. van Elk
9
6
7
8
Abstract The Groningen gas field is one of the largest in the world and has produced over 2000
9
billion m
3
of natural gas since the start of production in 1963. The first earthquakes linked to gas
10
production in the Groningen field occurred in 1991, with the largest event to date being a local
11
magnitude (M
L
) 3.6. As a result, the field operator is leading an effort to quantify the seismic
12
hazard and risk resulting from the gas production operations, including the assessment of
13
liquefaction hazard. However, due to the unique characteristics of both the seismic hazard and the
14
geological subsurface, particularly the unconsolidated sediments, direct application of existing
15
liquefaction evaluation procedures is deemed inappropriate in Groningen. Specifically, the depth-
16
stress reduction factor (r
d
) and the Magnitude Scaling Factor (MSF) relationships inherent to
17
existing variants of the simplified liquefaction evaluation procedure are considered unsuitable for
18
use. Accordingly, efforts have first focused on developing a framework for evaluating the
19
liquefaction potential of the region for moment magnitudes (M) ranging from 3.5 to 7.0. The
20
limitations of existing liquefaction procedures for use in Groningen and the path being followed
21
to overcome these shortcomings are presented in detail herein.
22
23
Keywords Liquefaction, liquefaction hazard, magnitude scaling factor, depth-stress reduction
24
factor, induced seismicity, Groningen gas field
25
26
1
Professor, Dept. of Civil and Environmental Engineering, Virginia Tech, Blacksburg, VA, USA (email:
rugreen@vt.edu)
2
Senior Research Investigator, Department of Civil and Environmental Engineering, Imperial College London,
London, UK
3
Professor, Dept. of Civil and Environmental Engineering, Virginia Tech, Blacksburg, VA, USA
4
Assistant Professor, Dept. of Civil and Environmental Engineering, University of Washington, Seattle, WA, USA
5
Reader, Dept. of Civil and Environmental Engineering, Imperial College London, London, UK
6
Senior Lecturer, School of Environmental Sciences, University of Liverpool, Liverpool, UK
7
Senior Geophysicist, Deltares, Delft, the Netherlands
8
Senior Engineering Geologist, Deltares, Delft, the Netherlands
9
Development Lead Groningen Asset, Nederlandse Aardolie Maatschappij B.V., Assen, the Netherlands

2
27
1 Introduction
28
29
The Groningen gas field is located in the northeastern region of the Netherlands and is one of the
30
largest in the world. It has produced over 2000 billon m
3
of natural gas since the start of production
31
in 1963. The first earthquakes linked to gas production in the Groningen field occurred in 1991,
32
although earthquakes were linked to production at other gas fields in the region since 1986. To
33
date the largest induced earthquake due to production at the Groningen field is the 2012 local
34
magnitude (M
L
) 3.6 Huizinge event, and the largest recorded peak ground acceleration (PGA) is
35
0.11 g which was recorded during a more recent, smaller (M
L
3.4) event. In response to concerns
36
about the induced earthquakes, the field operator Nederlandse Aardolie Maatschappij (NAM) is
37
leading an effort to quantify the seismic hazard and risk resulting from the gas production
38
operations (van Elk et al. 2017). In view of the widespread deposits of saturated sands in the region,
39
the risk due to earthquake-induced liquefaction is being evaluated as part of this effort. Although
40
an almost negligible contributor to earthquake fatalities, liquefaction triggering is an important
41
threat to the built environment and in particular to infrastructure and lifelines (e.g., Bird and
42
Bommer 2004).
43
44
Central to the liquefaction hazard/risk assessment of the Groningen field is the stress-based
45
“simplified” liquefaction evaluation procedure, which is the most widely used approach to evaluate
46
liquefaction potential worldwide. While most of the recently proposed variants of this procedure
47
yield similar results for scenarios that are well represented in the liquefaction case history
48
databases (e.g., Green et al. 2014), their predictions deviate, sometimes significantly, for other
49
scenarios (e.g., small and large magnitude events; very shallow and very deep liquefiable layers;
50
high fines content soils; medium dense to dense soils). These deviations result partly because
51
existing variants of the simplified procedure are semi-empirical, hence they are apt for replicating
52
existing data but lack proper extrapolation power. The empirical elements of existing procedures
53
are derived from data from tectonic earthquakes in active shallow-crustal tectonic regimes such as
54
California, Japan, and New Zealand. These conditions are different from those in the Groningen
55
field. Moreover, the geologic profiles/soil deposits in Groningen differ significantly from those
56
used to develop the empirical aspects of the simplified procedure. As a result, the suitability of
57

3
existing variants of the simplified procedure for direct use to evaluate liquefaction in Groningen
58
is questionable. Accordingly, prior to assessing the liquefaction hazard in Groningen, efforts have
59
first focused on developing a framework for performing the assessment. This actually required a
60
step backwards to develop an “unbiased” liquefaction triggering procedure for tectonic
61
earthquakes, due to biases in relationships inherent to existing variants of the simplified procedure
62
(e.g., Boulanger and Idriss 2014).
63
64
In the following sections, the shortcomings in current variants of the simplified procedures for use
65
in Groningen are detailed. Then, the efforts to develop a new “unbiased” variant of the simplified
66
liquefaction evaluation procedure are presented. An outline of how this procedure is being
67
modified for use in Groningen is presented next, followed by a brief overview of how the
68
liquefaction hazard of Groningen will be assessed.
69
70
2 Shortcoming in existing variants of the simplified liquefaction evaluation procedure for
71
use in Groningen
72
73
2.1 Overview of the simplified procedure
74
75
As mentioned in the Introduction, the stress-based simplified liquefaction evaluation procedure is
76
central to the approach adopted to assess the liquefaction hazard in the Groningen region. The
77
word “simplified” in the procedure’s title originated from the proposed use of a form of Newton’s
78
Second Law to compute cyclic shear stress (τ
c
) imposed at a given depth in the soil profile, in lieu
79
of performing numerical site response analyses (Whitman 1971; Seed and Idriss 1971). Inherent
80
to this approach for computing the seismic demand is an empirical depth-stress reduction factor
81
(r
d
) that accounts for the non-rigid response of the soil profile and a Magnitude Scaling Factor
82
(MSF) that accounts for the effects of the shaking duration on liquefaction triggering. For historical
83
reasons the duration of a moment magnitude (M) 7.5 earthquake is used as the reference for MSF.
84
85
Case histories compiled from post-earthquake investigations were categorized as either
86
“liquefaction” or “no liquefaction” based on whether evidence of liquefaction was or was not
87
observed. The seismic demand (or normalized Cyclic Stress Ratio: CSR*) for each of the case
88
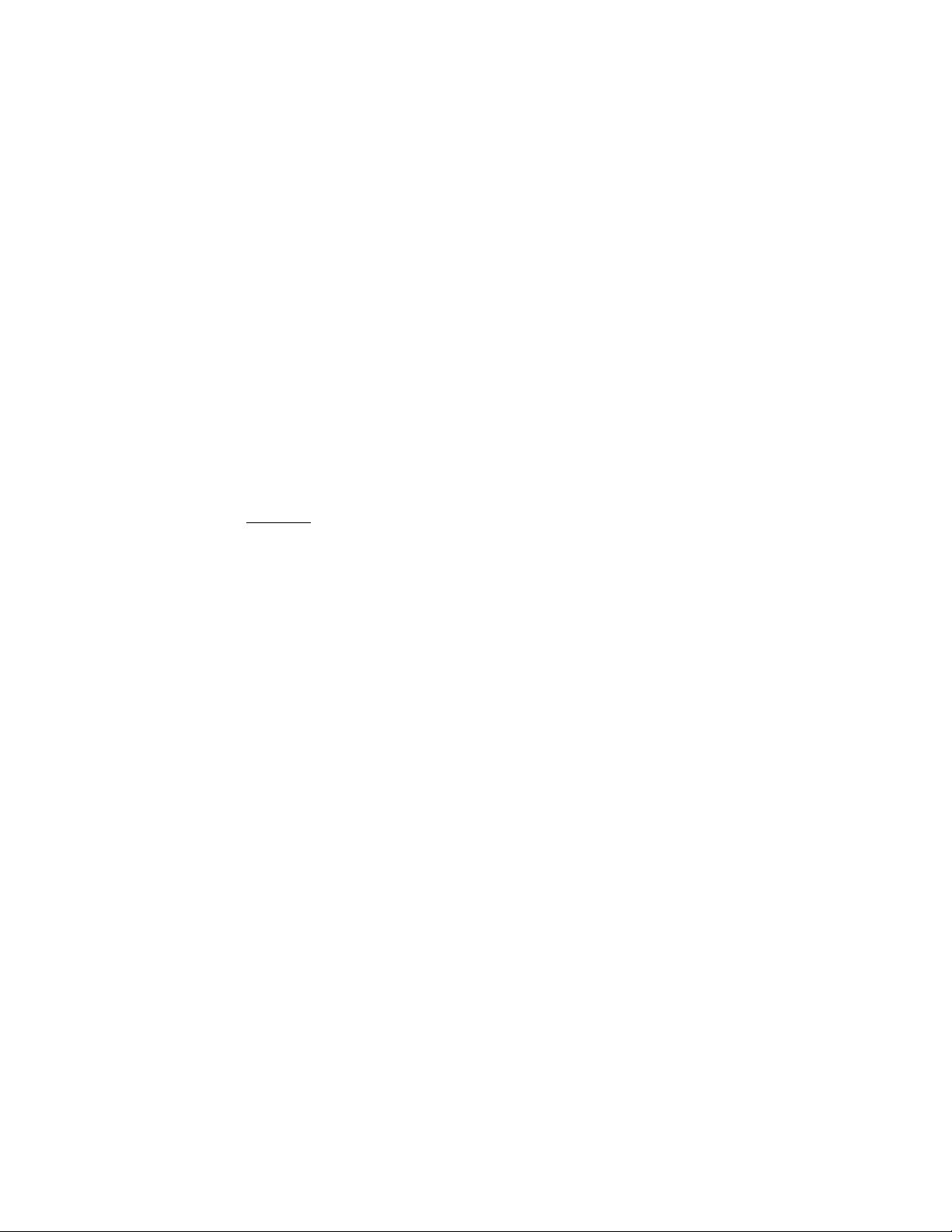
4
histories is plotted as a function of the corresponding normalized/fines-content corrected in situ
89
test metric, e.g., Standard Penetration Test (SPT): N
1,60cs
; Cone Penetration Test (CPT): q
c1Ncs
; or
90
small strain shear-wave velocity (V
S
): V
S1
. In this plot, the “liquefaction” and “no liquefaction”
91
cases tend to lie in two different regions of the graph. The “boundary” separating these two sets of
92
case histories is referred to as the Cyclic Resistance Ratio (CRR
M7.5
) and represents the capacity
93
of the soil to resist liquefaction during an M 7.5 event for level ground conditions and an effective
94
overburden stress of 1 atm. This boundary can be expressed as a function of the normalized in situ
95
test metrics.
96
97
Consistent with the conventional definition for factor of safety (FS), the FS against liquefaction
98
(FS
liq
) is defined as the capacity of the soil to resist liquefaction divided by the seismic demand:
99
100
(1)
101
The Dutch National Annex to the Eurocode for the seismic actions (i.e., NPR 9998 2017),
102
recommends the use of the Idriss and Boulanger (2008) variant of the simplified liquefaction
103
evaluation procedure, but allows other variants to be used if they are in line with the safety
104
philosophy of the NPR 9998-2017. As a result, the Idriss and Boulanger (2008) variant and the
105
updated variant (Boulanger and Idriss 2014) have been used in several liquefaction studies in
106
Gronginen, resulting in predictions of potentially catastrophic liquefaction effects that have severe
107
implications for buildings and for infrastructure such as dikes.
108
109
2.2 Depth-stress reduction factor: r
d
110
111
As stated above, r
d
is an empirical factor that accounts for the non-rigid response of the soil profile.
112
Both the Idriss and Boulanger (2008) and Boulanger and Idriss (2014) variants of the simplified
113
liquefaction evaluation procedure use an r
d
relationship that was developed by Idriss (1999). As
114
shown in Figure 1, the Idriss (1999) r
d
relationship is a function of earthquake magnitude and
115
depth, with r
d
being closer to one for larger magnitude events (note that r
d
= 1 for all depths
116
corresponds to the rigid response of the profile). This is because larger magnitude events have
117
longer characteristic periods and, hence, ground motions with longer wavelengths. As a result,
118

5
even a soft profile will tend to respond as a rigid body if the characteristic wavelength of the ground
119
motions is significantly longer than the overall thickness of the profile. Accordingly, the
120
correlation between earthquake magnitude and the frequency content of the earthquake motions
121
significantly influences the r
d
relationship. This raises questions regarding the appropriateness of
122
the Idriss (1999) relationship, which was developed using motions recorded during tectonic events,
123
for evaluating liquefaction potential in Groningen where the seismic hazard is dominated by
124
induced earthquakes having magnitudes less than M 5.
125
126
Another issue with the Idriss (1999) r
d
relationship is that it tends to predict overly high CSR*
127
values at depth in a soil profile for tectonic events. This bias is illustrated in Figure 1 and is
128
pronounced for depths between ~3 to 20 m below the ground surface. As a result, when used to
129
evaluate case histories to develop the CRR
M7.5
curves that are central to the procedure, the biased
130
r
d
relationship results in a biased positioning of the CRR
M7.5
curve. The significance of this issue
131
is mitigated to some extent when the same r
d
relationship used to develop the CRR
M7.5
curve is
132
also used in forward analyses (i.e., the bias cancels out). However, this will not be the case if
133
site/region-specific r
d
relationships are developed and used in conjunction with a CRR
M7.5
curve
134
that was developed using a “biased” r
d
relationship.
135
136
137
Fig. 1 The red, blue, and green lines were computed using the Idriss (1999) r
d
relationship for M
138
5.5, M 6.5, and M 7.5 events, respectively. The grey lines were computed by Cetin (2000) from
139