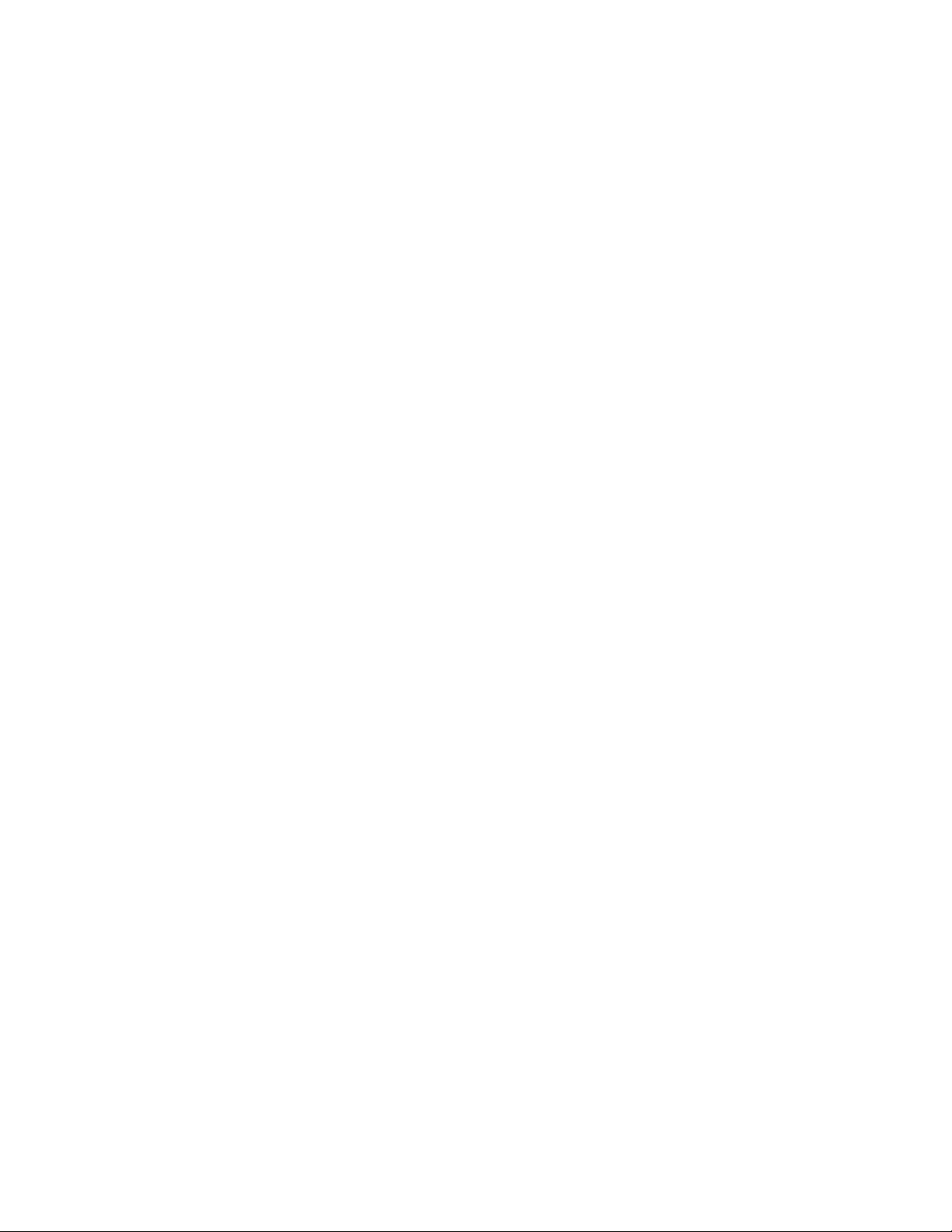
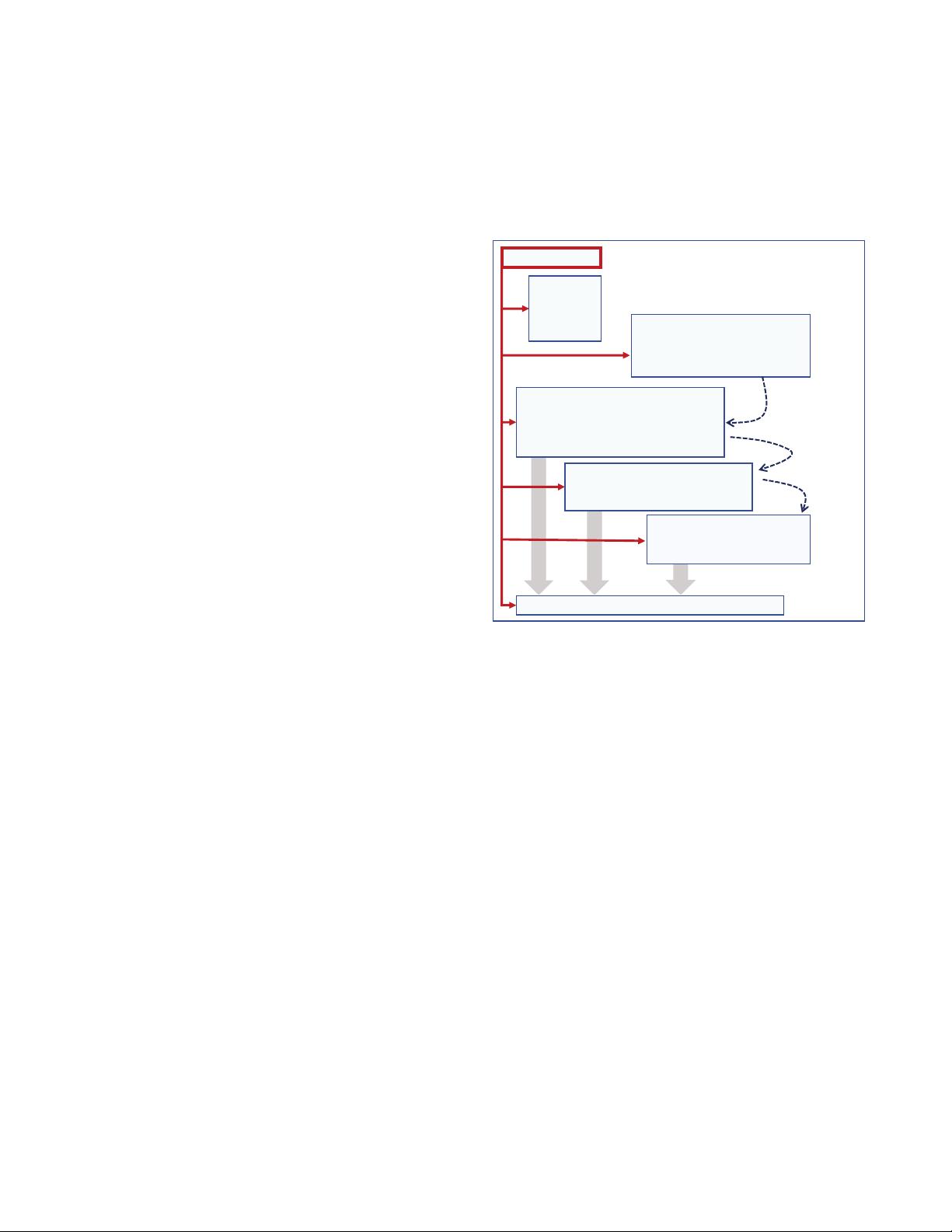
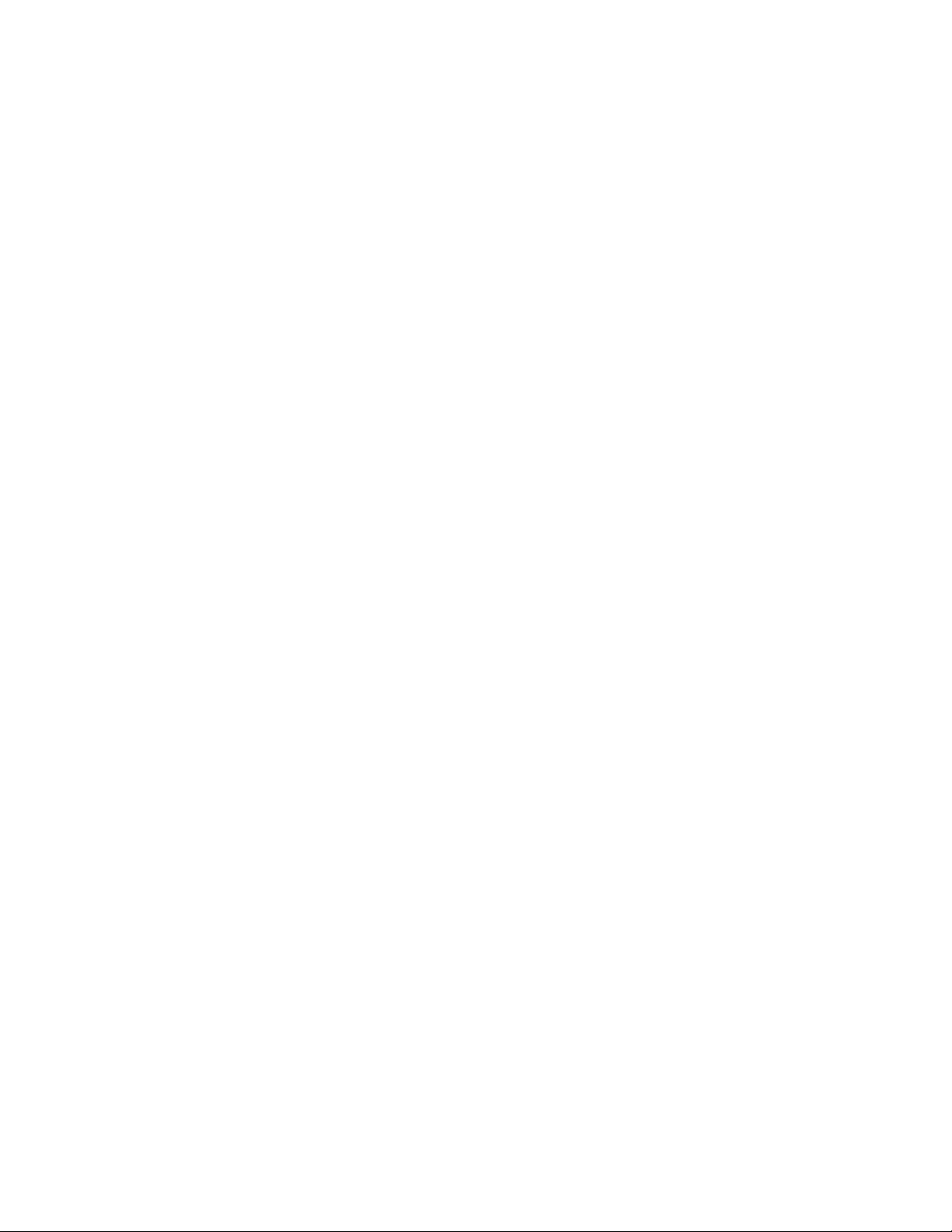


read more
It is also important to remark that accurate acquisition of instantaneous CSI is a key to fully exploiting large spatial9 diversity and multiplexing gains of mMIMO.
Suppose that a URLLC application aims to achieve an error probability of 10−5 and its required latency only allows a maximum K = 4 retransmissions in GF URLLC.
Emerging URLLC applications include virtual/augmented reality, public safety, factory automation, and autonomous vehicles, among others [7].
In order to further improve the spectral efficiency or shorten the latency of coded random access, a superposition of multiple orthogonal preambles [103] can be considered, which can effectively increase the number of active devices whose CSI can be estimated by the BS using SIC.
In particular, in random access, retransmissions are required as packet collisions are inevitable due to the nature of uncoordinated transmissions.
Since the estimated CSI is a noisy superposition of multiple channel vectors of the collided devices, it brings two effects in mMIMO, leading to unsuccessful decoding [89], [94]–[96]: 1) it reduces the coherent array gain of the desired received signal, and 2) it introduces a coherent interference that gets stronger as M grows.
with target reliability of = 10−5, it is evident that only 1 URLLC device can be served in MIMO with 8 antennas, while the number of URLLC devices that can be served simultaneously increases with M and can reach 55 when M = 128.
It is important to remark that the performance limitations for the HARQ retransmission schemes in GF random access are fundamentally originated from the multi-user interference as well as preamble collision when multiple devices compete for the same channel resource for uplink transmissions.
In GF URLLC, since multiple competing devices can transmit data over the same channel, it results in potential transmission collision, which is detrimental to the reliability.
it incurs additional latency and undesirable signalling overheads, which hinder achieving the required level of latency constraints for URLLC.
Earlier studies on the long-term evolution (LTE) systems to support URLLC [9] reported that LTE is not applicable to meet low-latency requirements.
with limited latency budget and wireless resources, their resulting reliability levels and spectral efficiency still needs to be enhanced to meet what is required for emerging URLLC services, particularly when the URLLC access load is relatively high [6].
As observed, with a fixed N , the decoding error probability can be significantly reduced as M grows, which reveals that the need for retransmissions can be dramatically reduced in GF URLLC.
In Fig. 3, a mini-slot based TTI of 21User-plane latency is the time it takes to successfully deliver a data packet at the radio protocol layer from the transmitter to the receiver.
Compared to the 4-step random access, GF random access can be more efficient thanks to low signaling overhead when devices have short packets to transmit.
Based on [14], [91], [92], the decoding error probability of receiving q bits of data within d channel uses can be well approximated by(γ) ≈ E{γ}[ Q ( d log2(1 + γ)− q√V (γ)d)] , (1)where E{x}[·] is the expectation operation over variable x, V (γ) is the channel dispersion that is given by V (γ) =( 1− 1(1+γ)2 ) log22(e).