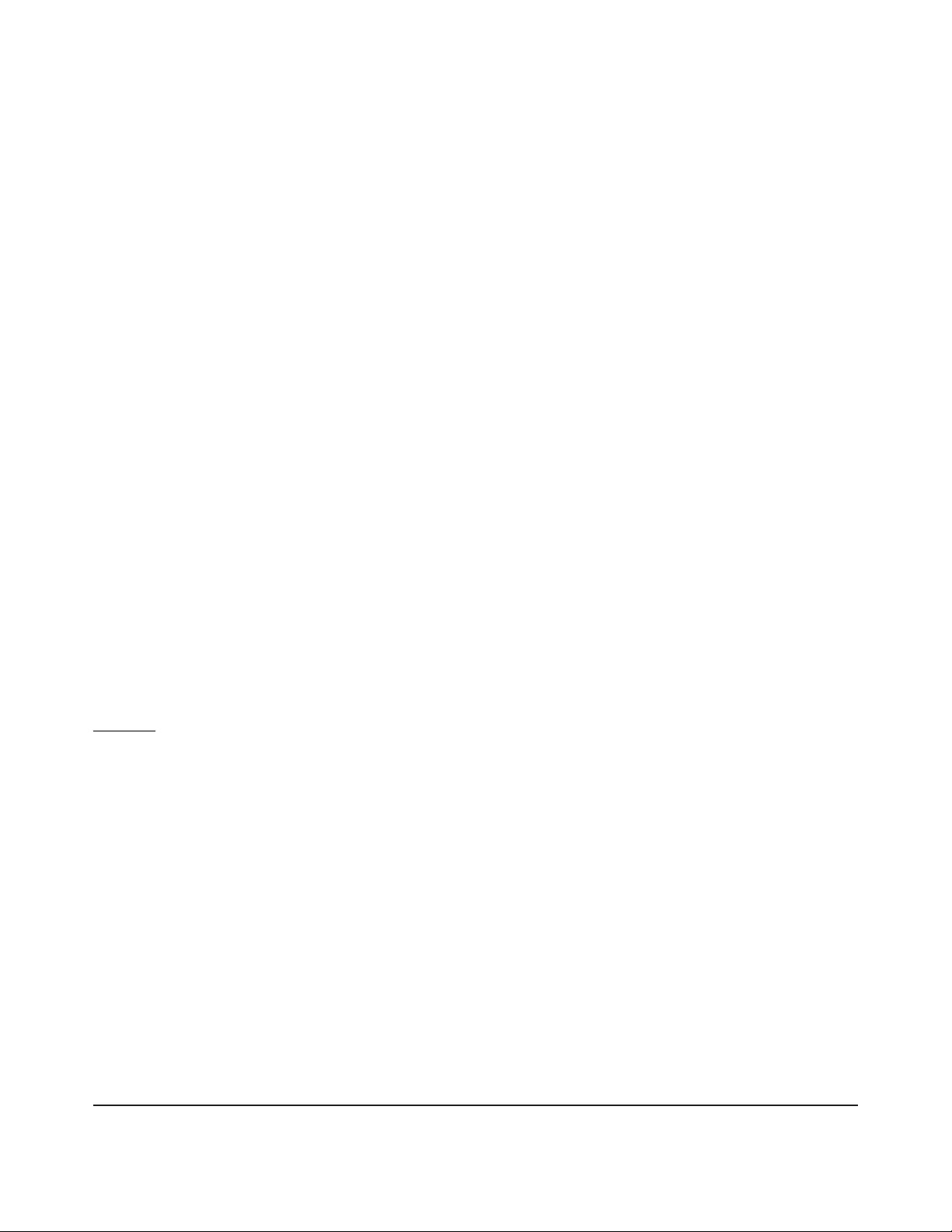
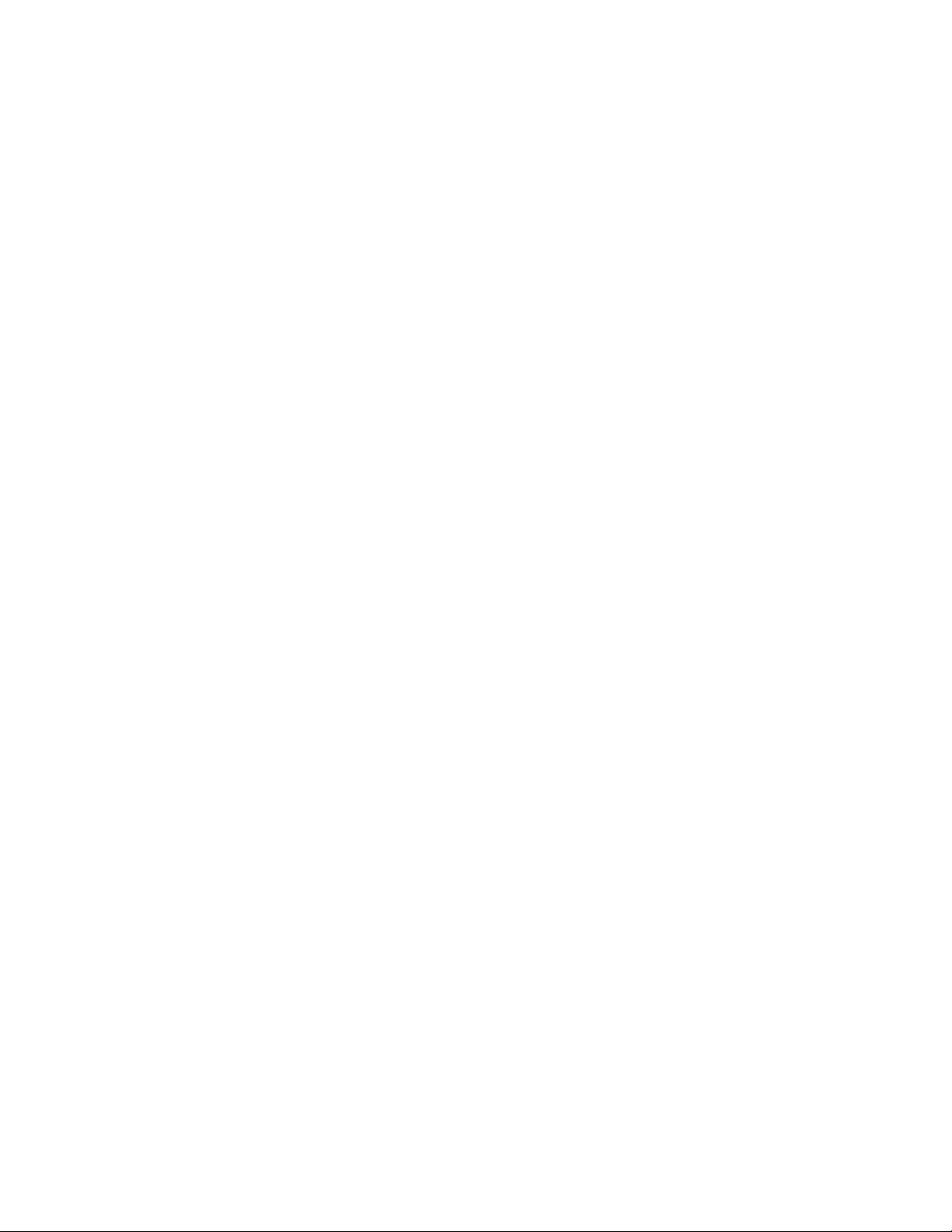
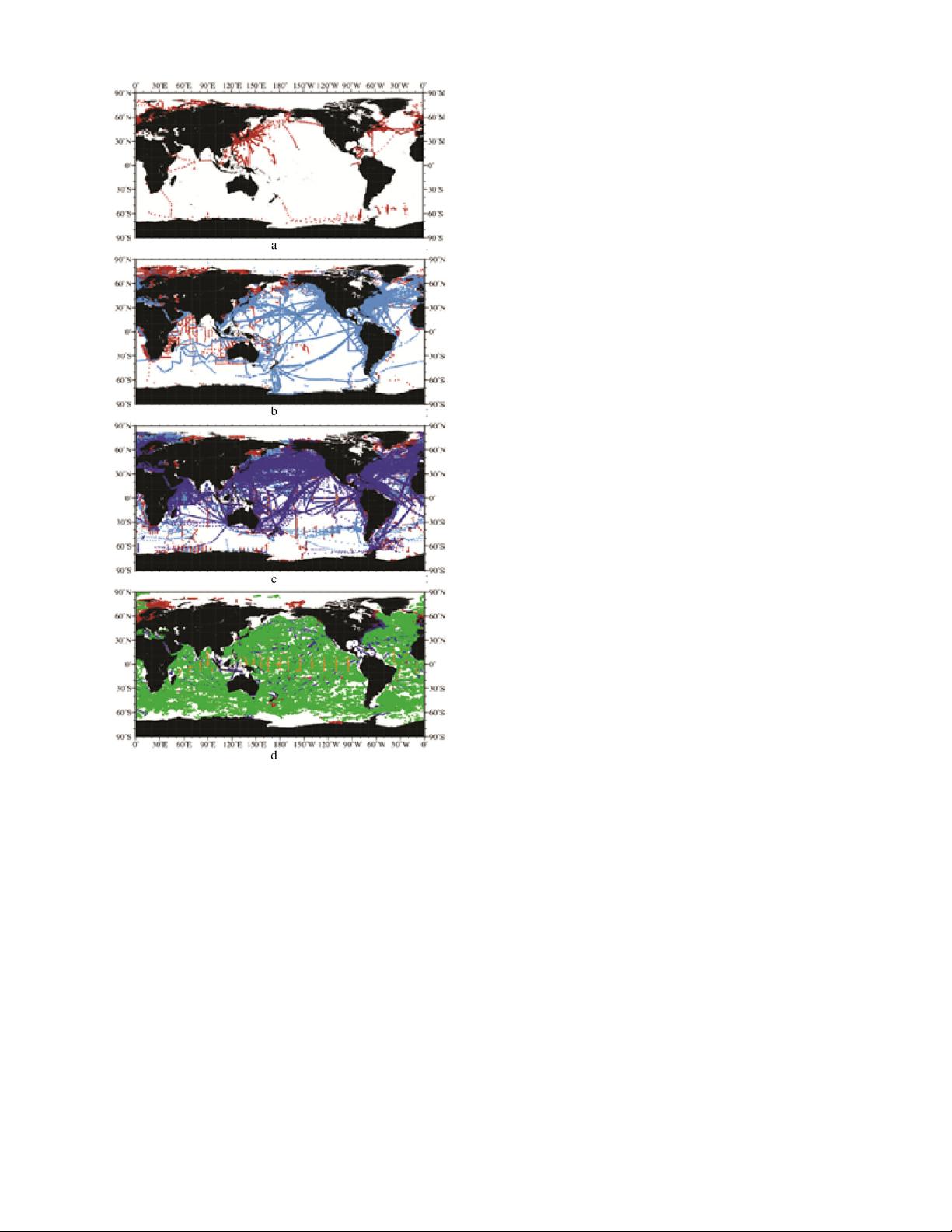


read more
Despite these potential future improvements to ocean monitoring, past and present measurements show that the Earth is experiencing a net gain in heat, largely from anthropogenic factors [ Hansen et al., 2005 ; Levitus et al., 2001 ], although the magnitude differs among individual studies.
Technological advances in wire and wire insulation made it possible to create an instrument electrically connected to the ship and able to transmit information through a thin conducting wire.
The expected lifetime of an Argo float is 3–5 years, so the fleet must be continually renewed to maintain the 3000 float goal. [28]
Modern shipboard CTD temperature sensors have a time response of 0.065 s (compared to 0.2–0.4 s for the MBT stylus), which allow the acquisition of accurate pressure/temperature profiles at a fairly rapid deployment rate from the surface to the deep ocean.
The Challenger was equipped with a pressure-shielded thermometer [Anonymous, 1870; Wollaston, 1782; Roemmich et al., 2012] to partially counteract the effects of pressure on temperature at great depths.
since it was an instrument that was mainly deployed from research ships, the CTD could not replace the MBT observing network.
With respect to sea level rise, mutually reinforcing information from tide gauges and radar altimetry shows that presently, sea level is rising at approximately 3mmyr 1 with contributions from both thermal expansion and mass accumulation from ice melt.
Pairs of protected and unprotected reversing thermometers were used to determine temperature and pressure, with pressure determined to an accuracy of ±5m depth in the upper 1000m.
On Captain James Cook’s second voyage (1772–1775), water samples were obtained from the subsurface Southern Ocean and it was found that surface waters were colder than waters at 100 fathoms (~183m) [Cook, 1777].
From 1967 to 2001, the XBT was a major contributor to the subsurface temperature observing system and was responsible for the growth of this system.
The array is important for local heat content calculations [e.g., Xue et al., 2012], and even the exclusion of one meridional set of buoys from the heat content calculation during the 1997–1998 El Niño led to a significant underestimate of heat content anomaly.
Those instruments (the expendable bathythermograph (XBT) and the Argo floats) are among the most important instruments for assessing ocean temperatures globally, and they provide up-to-date ocean subsurface temperature measurements.
By 1946, MBTs could reach to 900 feet (~275m), although the shallower version was deployed more often every year except 1964 (49% shallower version).
The XBT response time, at 0.15 s, is slower than modern shipboard CTDs, its accuracy likewise, at 0.15°C and 2% or 5m in depth, whichever is greater.
The problems during this time period with regard to a global ocean observing system were that Nansen bottle/reversing thermometer systems could only measure at a few discrete levels at each oceanographic station and that it was time consuming to deploy the instrumentation and make the measurements.
A stylus attached to the Bourdon tube captures the movement as temperature change horizontally scratched on a plate of smoked glass.