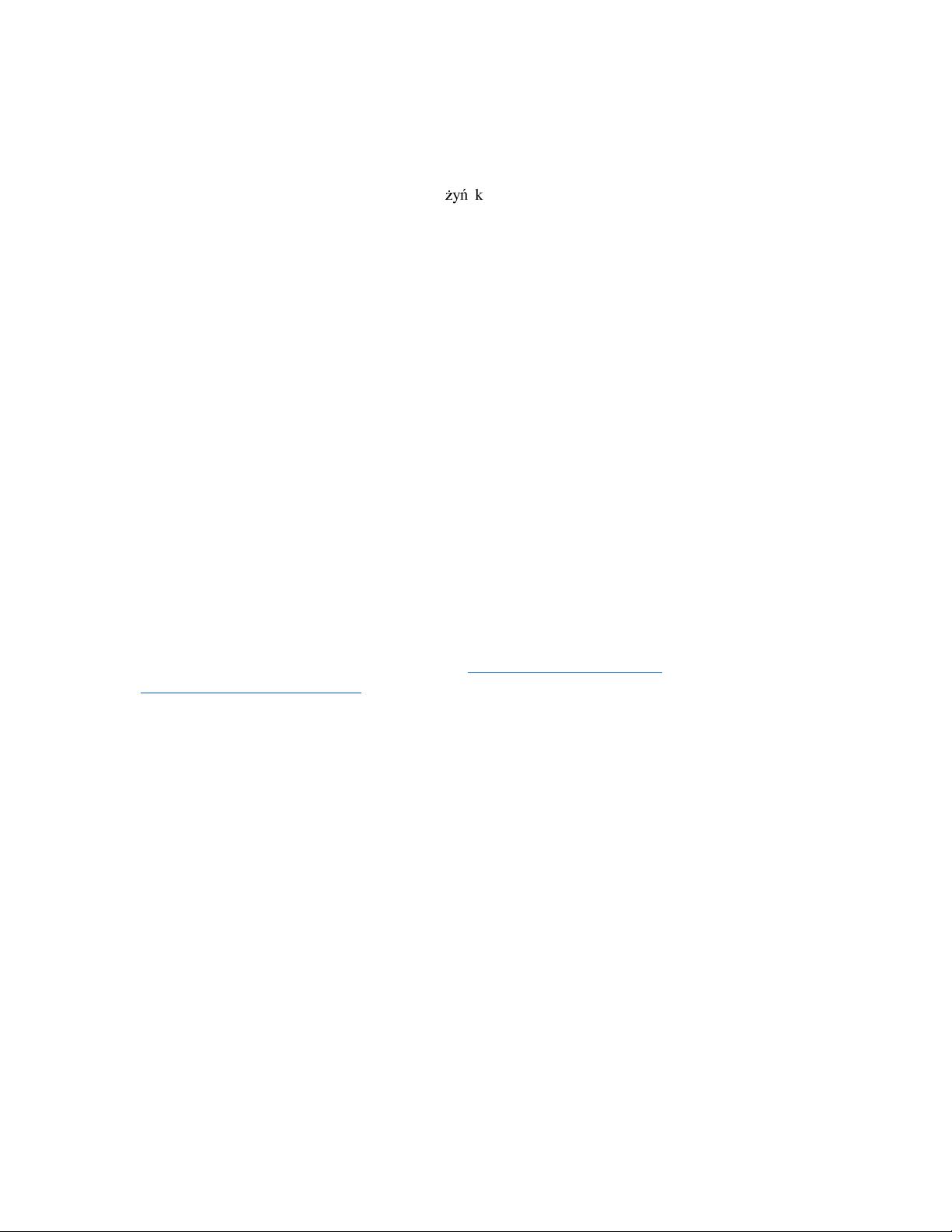
Combined effects of host genetics and diet on human gut microbiota and 1
incident disease in a single population cohort 2
3
Youwen Qin
1,2
, Aki S. Havulinna
3
, Yang Liu
1,4
, Pekka Jousilahti
3
, Scott C. Ritchie
1,5-7
, Alex Tokolyi
8
, 4
Jon G. Sanders
9,10
, Liisa Valsta
3
, Marta Bro
ż
y
ń
ska
1
, Qiyun Zhu
11
, Anupriya Tripathi
11,12
, Yoshiki 5
Vazquez-Baeza
13,14
, Rohit Loomba
15
, Susan Cheng
16
, Mohit Jain
11,13
, Teemu Niiranen
3,17
, Leo Lahti
18
, 6
Rob Knight
11,13,14
, Veikko Salomaa
3
, Michael Inouye
1,2,5-7,19-21*
§, Guillaume Méric
1,22*
§ 7
8
1
Cambridge Baker Systems Genomics Initiative, Baker Heart and Diabetes Institute, Melbourne, Victoria, 9
Australia;
2
School of BioSciences, The University of Melbourne, Melbourne, Victoria, Australia;
3
Department of 10
Public Health Solutions, Finnish Institute for Health and Welfare, Helsinki, Finland;
4
Department of Clinical 11
Pathology, The University of Melbourne, Melbourne, Victoria, Australia;
5
Cambridge Baker Systems Genomics 12
Initiative, Department of Public Health and Primary Care, University of Cambridge, UK;
6
British Heart 13
Foundation Centre of Research Excellence, University of Cambridge, UK;
7
National Institute for Health Research 14
Cambridge Biomedical Research Centre, University of Cambridge and Cambridge University Hospitals, 15
Cambridge, UK;
8
Wellcome Sanger Institute, Wellcome Genome Campus, Hinxton, UK;
9
Department of Ecology 16
and Evolutionary Biology, Cornell University, Ithaca, NY, USA;
10
Cornell Institute for Host-Microbe Interaction 17
and Disease, Cornell University, Ithaca, NY, USA;
11
Department of Pediatrics, School of Medicine, University of 18
California San Diego, La Jolla, CA, USA;
12
Division of Biological Sciences, University of California San Diego, 19
La Jolla, California, USA;
13
Center for Microbiome Innovation, University of California San Diego, La Jolla, CA, 20
USA;
14
Department of Computer Science & Engineering, Jacobs School of Engineering, University of California 21
San Diego, La Jolla, CA, USA;
15
NAFLD Research Center, Department of Medicine, University of California San 22
Diego, La Jolla, CA, USA;
16
Smidt Heart Institute, Cedars-Sinai Medical Center, Los Angeles, CA, USA; 23
17
Department of Medicine, Turku University Hospital and University of Turku, Turku, Finland;
18
Department of 24
Future Technologies, University of Turku, Turku, Finland;
19
British Heart Foundation Cardiovascular 25
Epidemiology Unit, Department of Public Health and Primary Care, University of Cambridge, UK;
20
Health Data 26
Research UK Cambridge, Wellcome Genome Campus & University of Cambridge, UK;
21
The Alan Turing 27
Institute, London, UK;
22
Department of Infectious Diseases, Central Clinical School, Monash University, 28
Melbourne, Victoria, Australia.
29
30
§ These authors contributed equally 31
*Corresponding authors: Michael Inouye: mi336@medschl.cam.ac.uk
; Guillaume Méric: 32
guillaume.meric@baker.edu.au
. 33
34
. CC-BY 4.0 International licenseIt is made available under a
is the author/funder, who has granted medRxiv a license to display the preprint in perpetuity.(which was not certified by peer review)preprint
The copyright holder for thisthis version posted September 13, 2020. ; https://doi.org/10.1101/2020.09.12.20193045doi: medRxiv preprint
NOTE: This preprint reports new research that has not been certified by peer review and should not be used to guide clinical practice.

Abstract 35
36
Co-evolution between humans and the microbial communities colonizing them has resulted in 37
an intimate assembly of thousands of microbial species mutualistically living on and in their 38
body and impacting multiple aspects of host physiology and health. Several studies examining 39
whether human genetic variation can affect gut microbiota suggest a complex combination of 40
environmental and host factors. Here, we leverage a single large-scale population-based cohort 41
of 5,959 genotyped individuals with matched gut microbial shotgun metagenomes, dietary 42
information and health records up to 16 years post-sampling, to characterize human genetic 43
variations associated with microbial abundances, and predict possible causal links with various 44
diseases using Mendelian randomization (MR). Genome-wide association study (GWAS) 45
identified 583 independent SNP-taxon associations at genome-wide significance (p<5.0×10
-8
), 46
which included notable strong associations with LCT (p=5.02×10
-35
), ABO (p=1.1×10
-12
), and 47
MED13L (p=1.84×10
-12
). A combination of genetics and dietary habits was shown to strongly 48
shape the abundances of certain key bacterial members of the gut microbiota, and explain their 49
genetic association. Genetic effects from the LCT locus on Bifidobacterium and three other 50
associated taxa significantly differed according to dairy intake. Variation in mucin-degrading 51
Faecalicatena lactaris abundances were associated with ABO, highlighting a preferential 52
utilization of secreted A/B/AB-antigens as energy source in the gut, irrespectively of fibre 53
intake. Enterococcus faecalis levels showed a robust association with a variant in MED13L, 54
with putative links to colorectal cancer. Finally, we identified putative causal relationships 55
between gut microbes and complex diseases using MR, with a predicted effect of Morganella 56
on major depressive disorder that was consistent with observational incident disease analysis. 57
Overall, we present striking examples of the intricate relationship between humans and their 58
gut microbial communities, and highlight important health implications. 59
60
. CC-BY 4.0 International licenseIt is made available under a
is the author/funder, who has granted medRxiv a license to display the preprint in perpetuity.(which was not certified by peer review)preprint
The copyright holder for thisthis version posted September 13, 2020. ; https://doi.org/10.1101/2020.09.12.20193045doi: medRxiv preprint

Introduction 61
62
Humans have co-evolved with the microbial communities that colonize them, resulting in a 63
complex assembly of thousands of microbial species mutualistically living in their 64
gastrointestinal tract. A fine-tuned interplay between microbial and human physiologies can 65
impact multiple aspects of development and health to the point that dysbiosis is often 66
associated with disease
1–3
. As such, increasing evidence points to the influence of human 67
genetic variation on the composition and modulation of their gut microbiota. 68
69
Past genetic studies have collectively revealed important host-microbe interactions
4–14
. 70
Previous twin studies detected significant heritability signal from the presence and abundance 71
of only a few microbial taxa, such as some Firmicutes
15
, suggesting a strong transientness and 72
variability in gut microbial composition, as well as an important influence from external 73
factors
6,15–18
. Nonetheless, a well-described association between Bifidobacterium levels and 74
LCT-MCM6, governing the phenotype of lactase persistence throughout adulthood in 75
Europeans, was uncovered in 2015
4
and subsequently replicated by later studies
6,7,9–12
, 76
suggesting a very strong influence of the evolution of dairy diet in modern humans on their gut 77
bacteria. Additionally, genes involved in immune and metabolic processes
9
but also disease
19
78
were also associated with gut microbial variation. Despite several promising findings, 79
reproducibility across studies varying in sampling and methods is generally poor, and most 80
previously reported associations lose significance after multiple testing corrections
20
. The 81
individual gut microbiota is largely influenced by environmental variables, mostly diet and 82
medication
21–23
, which could explain a larger proportion of microbiome variance than 83
identifiable host genetic factors
9,10
. Biological factors could also influence the cross-study 84
reproducibility of results. GWAS would typically not reproducibly identify genetic 85
associations with taxa harbouring microbial functions potentially shared by multiple unrelated 86
species
24,25
. Indeed, a certain degree of functional redundancy has been observed in human gut 87
microbial communities
25
, which is believed to play a role in the resistance and resilience to 88
perturbations
26–28
. However, both assembly and functioning in human gut microbial 89
communities seem to be driven by the presence of a few particular and identifiable keystone 90
taxa
29
, which exert key ecological and modulatory roles on gut microbial composition 91
independently of their abundance
30,31
. Such taxa are relatively prevalent across individuals and 92
thought to be part of the human “core” microbiota
30,31
, which makes them potentially 93
identifiable through GWAS. 94
95
Increasing sample size in studied populations could yield novel and robustly associated results, 96
and alleviate the effect of confounding technical or biological factors. This could be achieved 97
either by performing meta-analyses of GWAS conducted in various populations
12
, or by using 98
larger cohort datasets. In this study, we used a large single homogenous population cohort with 99
matching human genotypes and shotgun faecal metagenomes (N=5959; FINRISK 2002 100
(FR02)) to identify novel genome-wide associations between human genotypes and gut 101
microbial abundances (Figure S1). We further leveraged additional and extensive health 102
registry and dietary individual data to investigate the effects of diet and genotype on particular 103
host-microbial associations, and to predict incident disease linked to gut microbial variation. 104
105
Results
106
107
Genome-wide association analysis of gut microbial taxa 108
109
Genome-wide association tests were applied to 2,801 microbial taxa and 7,979,834 human 110
genetic variants from 5,959 individuals enrolled in the FR02 cohort, which includes all taxa 111
. CC-BY 4.0 International licenseIt is made available under a
is the author/funder, who has granted medRxiv a license to display the preprint in perpetuity.(which was not certified by peer review)preprint
The copyright holder for thisthis version posted September 13, 2020. ; https://doi.org/10.1101/2020.09.12.20193045doi: medRxiv preprint
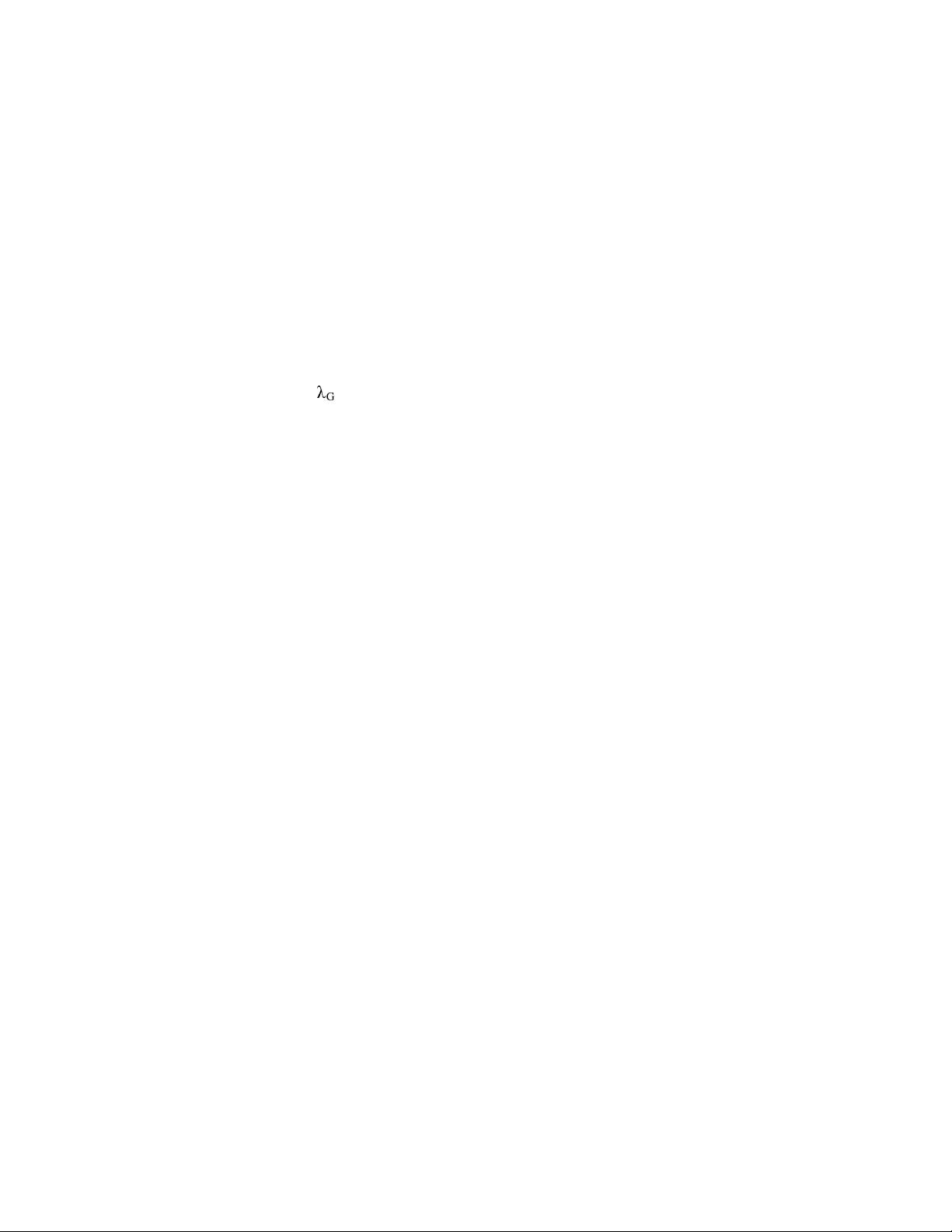
discovered to be prevalent in >25% of the cohort (Methods). Using a genome-wide 112
significance threshold (p<5.0×10
-8
), a total of 478 distinct GTDB taxa, which represented 17% 113
of all tested taxa and included 11 phyla, 19 classes, 24 orders, 63 families, 148 genera and 213 114
species, were found to be associated with at least one genetic variant (Figure 1, Table S1). 115
Conditional analysis found 583 independent SNP-taxon associations at genome-wide 116
significance (Table S1). Heritability across the 2,801 taxa ranged between h
2
=0.001 to 0.214, 117
with the highest values observed for taxa belonging to the Firmicutes and Firmicutes_A GTDB 118
phyla, both of which encompassed half (241/476, 50.4%) of all associated taxa with genetic 119
variation (Figure S2). There were no differences in SNP heritability between groups of 120
associated or non-associated taxa at genome-wide significance (p=0.23). 121
122
Three loci were strongly associated with microbial variation at study-wide significance, as 123
shown on a Manhattan plot showing the lowest resulting p-value for each SNP tested against 124
each of the 2,801 taxa (Figure 1, Table 1). There was no evidence of excess false positive rate 125
in the GWAS (median
λ
GC
=1.0051) (Figure 1B). After conditional analysis, the strongest 126
association by far (p=5.0×10
-35
) involved members of class Actinobacteria and rs3940549, a 127
variant in the LCT-MCM6-ZRANB3 locus region which is in high LD (r
2
=0.87) with the well-128
described LCT variant rs4988235 causing lactase persistence in adults of European ancestry 129
(Figure S3). In total, 29 taxa were associated with the LCT-MCM6 region, including 18 below 130
study-wide significance (Figure 1, Table S1). These involved Bifidobacterium-related 131
Actinobacteriota and three taxa from the GTDB Firmicutes_A phylum which included 2 132
uncultured species defined from metagenome-assembled reference genomes (UBA3855 133
sp900316885 and CAG-81 sp000435795) (Table 1). The association of these three 134
Firmicutes_A with LCT was still genome-wide significant after adjusting for Bifidobacterium 135
abundances (Table S2). A variant in ABO (rs545971), expressing the histo-blood 136
group ABO system transferase, was strongly associated (p=1.1×10
-12
) with levels of 137
Faecalicatena lactaris. There was evidence for a second independent signal at ABO associated 138
with the Collinsella genus (chr9:133271182; p=2.5×10
-8
) (Table S1, Figure 1). Rs187309577 139
and rs143507801 in MED13L, expressing the Mediator complex subunit 13L, were found to be 140
associated with genus Enterococcus (p=1.8×10
-12
) and the Enterococcus faecalis species 141
(p=7.26×10
-11
), respectively (Table S1, Figure 1). 142
143
Human gut microbiome keystone taxa are associated with genetic variation 144
145
In total, we identified 31 distinct genetic variants associated (p<5.0×10
-8
) with 39 microbial 146
taxa related to identified keystone species as listed by Banerjee et al. (2018)
29,32
, which 147
included the Actinobacteria class
30
, Helicobacter pylori
29
, Bacteroides stercoris
33
, Bacteroides 148
thetaiotaomicron
34
, Ruminococcus bromii
35
, Klebsiella pneumoniae
36
, Proteus mirabilis
36
, 149
Akkermansia muciniphila
31
, and the archaeon Methanobrevibacter smithii
37,38
(Figure 1C, 150
Table S1). Only one documented keystone species from Banerjee et al.
29
, Bacteroides 151
fragilis
39
, was not associated with genetic variation in our study. This observation suggests that 152
keystone species, although defined as exerting selective modulation and not broad effects on 153
microbiome composition variation, generally associates with human genetic variation, 154
suggesting an intimate association with the human gut niche, in line with their reported key 155
ecological roles in microbiome modulation and functioning. Our work highlights novel human 156
genotypes possibly associated with keystone taxa (Table S1), which could further improve our 157
understanding of their ecology. 158
159
Combined effect of host genetics and dietary dairy intake on gut levels of LCT-associated 160
bacteria 161
162
. CC-BY 4.0 International licenseIt is made available under a
is the author/funder, who has granted medRxiv a license to display the preprint in perpetuity.(which was not certified by peer review)preprint
The copyright holder for thisthis version posted September 13, 2020. ; https://doi.org/10.1101/2020.09.12.20193045doi: medRxiv preprint

We compared the abundances of 4 bacterial taxa strongly associated with the LCT locus 163
(Bifidobacterium genus, Negativibacillus genus, UBA3855 sp900316885 and CAG-81 164
sp000435795) in individuals with different rs4988235 genotypes and dairy diets (Figure 2A). 165
The abundance of Bifidobacterium in individuals producing lactase through adulthood 166
(rs4988235:TT) was unaffected by dairy intake. However, lactose-intolerant individuals 167
(rs4988235:CC) self-reporting a regular dairy diet had a significant increase in Bifidobacterium 168
abundance (p=1.75×10
-13
; Wilcoxon-rank test). An intermediate genotype (rs4988235:CT) was 169
linked to an intermediate increase (Figure 2A). This trend did not seem to be affected by age
40
170
(Figure S4). 171
172
An inverse pattern was observed for the abundance distributions of Negativibacillus and 173
uncultured CAG-81 sp000435795, for which abundances decreased in lactose intolerant 174
individuals reporting dairy intake, as compared to rs4988235:TT individuals consuming dairy 175
products (p=0.049 and p=0.041, respectively) (Figure 2A). Levels of UBA3855 sp900316885 176
were unaffected by a dairy diet in lactose-intolerant individuals but were surprisingly lower in 177
rs4988235:TT individuals who reported dairy intake (p=8.23×10
-5
) (Figure 2A). These 178
opposite and contrasting effects of dairy intake on associated bacterial abundances in lactose-179
intolerant individuals could reflect competition for lactose in the gut. Genus CAG-81 180
abundances were the most negatively correlated with those of the other LCT-associated taxa 181
(Figure S5), which suggests that this competition could be strong and prevalent enough to 182
drive co-association at the LCT locus, possibly mediated by lactose intake (Figure 2B). 183
184
Functional profiling of CAZymes in 11 Bifidobacterium species 185
186
Of all 11 Bifidobacterium species prevalent enough in our study population to be included in 187
the GWAS, only B. dentium was not associated with the LCT locus (p=1.70×10
-2
), nor was it 188
co-abundant with any other Bifidobacterium species (Figure S6A). B. dentium has previously 189
been suggested to have different metabolic abilities
41
. A clustering of carbohydrate-active 190
enzymes (CAZyme) profiles from reference genomes of all 11 Bifidobacterium species 191
revealed that B. dentium clustered apart from the 10 other species, which grouped consistently 192
with their co-abundance patterns (Figure S6B). B. dentium harboured more genes encoding 193
CAZyme families with preferred fiber/plant-related substrates (GH94, GH26, GH53) than 194
other Bifidobacterium species, which seemed to harbour more milk oligosaccharide-targeting 195
CAZyme families (GH129, GH112) than B. dentium (Figure S6B), which could relate to the 196
observed association differences. This suggests that bacterial metabolic abilities can be strong 197
drivers of co-abundance, and of association with human genetic variation. 198
199
Functionally distinct ABO-associated bacteria are impacted differently by genotype and 200
dietary fiber intake 201
202
A variety of bacteria metabolize blood antigens, with potential applications in synthetic 203
universal donor blood production
42,43
. Gut bacteria are particularly exposed to A- and B-204
antigens in the gut mucosa of secretor individuals
44
. Our associations of Faecalicatena lactaris 205
(p=1.10×10
-12
) and Collinsella (p=2.59×10
-8
) with ABO suggest a possible metabolic link with 206
blood antigens. A comparison of CAZyme profiles across a set of reference genomes revealed 207
3 CAZymes with blood-related activities in F. lactaris (GH110
45
, GH136
46
, CBM32
47
), but 208
none in any of 9 Collinsella species (Figure 3A). More mucus-targeting and less fiber-209
degrading enzymes were found in F. lactaris than Collinsella (Figure 3A), suggesting distinct 210
functions in the gut. 211
212
. CC-BY 4.0 International licenseIt is made available under a
is the author/funder, who has granted medRxiv a license to display the preprint in perpetuity.(which was not certified by peer review)preprint
The copyright holder for thisthis version posted September 13, 2020. ; https://doi.org/10.1101/2020.09.12.20193045doi: medRxiv preprint